For further reading, please check out our blog series covering the constraints, risks, and opportunities revealed by this analysis.
Summary for Policymakers: Analysis of Net-Zero Pathways for the EU and UK
Overview
The European Union (EU) and United Kingdom (UK) have set ambitious clean energy and emission reduction goals. This includes the European Green Deal committing the EU to carbon neutrality by 2050 and a 55% reduction in greenhouse gas (GHG) emissions by 2030 (with the Fit for 55 package under consideration to achieve the 2030 goals), and the UK’s 78% GHG reduction target by 2035. These proposals, however, leave a lot of flexibility for how to cut emissions in that timeframe. They also leave open how the EU and UK will get to net-zero in less than three decades, putting off much of the essential planning for clean energy technologies and supportive infrastructure that will be necessary to fully decarbonise.
Carbon-Free Europe (CFE) conducted a modelling analysis to identify five different pathways for the EU and UK to reach their commitment of net-zero emissions by 2050. This analysis builds on existing decarbonisation modelling efforts to explore the opportunities, risks, and additional constraints Europe and individual countries face to reach carbon neutrality. Our results show crucial steps Europe must take over the next three decades to ensure credible trajectories to net-zero. The analysis reinforces that the chances of reaching emissions goals for individual countries and the EU increase as more clean technology and fuel options are included as viable mitigation options. Increased optionality reduces risk and makes it more likely that clean energy will be cost-effective and net-zero goals can actually be achieved.
In this summary, we present topline modelling takeaways and discuss implications of our analysis for EU policymakers before providing a more in-depth explanation of our analysis and aforementioned takeaways. A more detailed technical powerpoint can be read here. In-depth results for each country can be found here. Interactive maps showing changes in Europe’s energy system including electric capacity, transmission, hydrogen infrastructure, and more are here. We provide open access to our results so that policymakers, analysts, and the public can evaluate the benefits and challenges for themselves.
Topline Takeaways
- Europe can achieve net-zero emissions by 2050 with a variety of potential energy mixes. Each net-zero pathway depends on different economic, political, social, and technical risks.
- Risk assessment is an important framework to use when evaluating long-term energy plans. Required costs as well as pace and scale of infrastructure transformation are two of the largest risks to the feasibility of net-zero pathways.
- The scale of electricity generation required to decarbonise Europe is enormous. Our model suggests that the amount of electricity generation will more than triple over the next 30 years as electrification of transport, heat, and hydrogen drives new demands for clean electricity.
- Decarbonisation changes the map of energy in Europe as the nature of energy resource endowments change. Whereas prior energy advantages included access to oil, natural gas, and coal resources, now resource advantages include: access to high quality renewable resources, biomass availability, geologic advantages for CO2 sequestration and hydrogen storage, and an acceptance of nuclear technology.
- Electrification of end-uses supported by the rapid and sustained deployment of renewables is responsible for a large fraction of the emissions reductions through 2030 and plays an important role to 2050. However, achieving net-zero emissions will require the deployment of other technologies.
EU Policy Implications
This analysis is in line with the Commission’s proposals under the Fit for 55 package to achieve 55% reduction in GHG emissions by 2030 and can inform the finalisation of key legislative proposals including: the Emission Trading System (ETS), Effort Sharing Regulation (ESR), the Renewable Energy Directive (RED II), the Energy Efficiency Directive, and others. Our modelling shows the range of deployment of different clean energy technologies necessary to meet climate goals for each Member State, demonstrates the impact of a carbon floor price rising to €108 by 2030, shows how countries can work together to transfer electricity and hydrogen across borders to major load centres, and reinforces the importance of electrifying buildings, surface transportation, and parts of industry.
Some governments are considering the reintroduction of coal and the increased use of other fossil fuels like gas as a transitional solution in light of Russia’s invasion of Ukraine. Our modelling shows the EU can still meet its energy requirements without a regressive turn back to fossil fuels, enabling the bloc to stick to its net-zero strategy. In order to do so, our results show the EU must speed up the deployment of renewable energy sources as well as reassess its stance on other carbon-free energy sources that are at risk of being phased out prematurely.
Beyond the 2030 timeline, our analysis can inform critical decision-making to achieve the European Green Deal objectives and ensure climate neutrality by 2050. The EU should carry out a thorough risk assessment when evaluating its long-term climate and energy plans. Required costs, pace, and scale of infrastructure transformation are important elements that impact the feasibility of these plans. In order to achieve net-zero in the most cost-effective way, our analysis shows that all carbon-free technologies must be treated equally at the EU level with regards to the regulatory frameworks and incentives for investing in these technologies.
At the Member State level, our modelling can inform and contribute to possible implications, risks, and opportunities in each country’s continual assessment and implementation of its National Energy and Climate Plans (NECP). Our analysis shows viable net-zero energy mixes for each country as well as opportunities to import or export energy, which can help inform national strategies. Further research from CFE will do a deeper dive to assess potential policies and technologies for individual Member States to reach carbon-neutrality.
About the Model
To conduct our analysis, we used a combination of two models developed by Evolved Energy Research (EER): the Regional Investment and Operations (RIO) supply-side model and EnergyPATHWAYS demand-side model. The RIO modelling platform optimises Europe’s energy supply over the coming three decades, with a high level of spatial and temporal resolution. Supply-side options include all existing energy technologies and innovative clean energy technologies that we anticipate will come down in costs and be more widely deployed in the coming decades. The model is able to show country-level results for all energy forms (electricity, heat, hydrogen, gas, refined fuels, etc.) and options for the transport of energy across Europe (electricity transmission lines, hydrogen pipelines, and other fuel delivery networks between countries). To ensure high temporal resolution, the analysis incorporates hourly resolution in both electricity as well as other energy products. This more accurately demonstrates low-cost and reliable energy systems and highlights the sector coupling opportunities that aid in operating high renewable power systems. For more about the models, read here.
Incorporating Resource Constraints
We explicitly incorporated resource constraints in our analysis because these constraints can become crucial limiting factors in a deeply decarbonised world. Oftentimes this consideration becomes the largest disconnect between a modelled and experienced reality. These constraints take the form of available land, open sea, or rooftop capacity for renewable energy deployment, availability of low-carbon biomass feedstocks, geologic sequestration potential, hydrogen salt cavern storage, and new electricity interconnections. To do so, we leverage significant primary data research conducted by and for the EU including work conducted for the Joint Research Centre (JRC). In addition, we developed our own renewable resource supply curves (solar, onshore wind, and offshore wind) for all the countries included in the analysis. These include resource constraints as well as resource quality disaggregation and necessary interconnection costs to connect resources to loads. For more on our analysis of resource constraints for renewables, read here.
Comparison with European Commission Modelling
Our findings in 2030 are very consistent with EU-level modelling conducted by the European Commission to support the EU’s Fit for 55 package. Most of the emissions reductions in the near to medium-term are achieved with the rapid deployment of renewables and electrification. In our modelling, we show several scenarios exceeding the emissions targets established in that exercise. This is accomplished with the acceleration of electrification, renewable deployment, and increased nuclear generation in 2030 (from existing and new plants). All of this acceleration is consistent with the increased imperative for Europe to reduce oil and natural gas usage. After 2030, the modelling stays in line with the EU and UK’s objective of reaching carbon neutrality by 2050, and demonstrates the breadth of potential energy futures that Europe may choose to meet its emissions targets.
Descriptions of Five Net-Zero Pathways
We analysed five different pathways, also referred to as scenarios, for the EU and UK to reach net-zero emissions by 2050: 1) Core, 2) Slow Demand Transformation, 3) 100% Renewable Primary Energy, 4) Limited Renewable Siting, and 5) Domestic Preference. All pathways achieve a 55% reduction from 1990 levels in energy and industrial emissions (for the EU and UK) by 2030 and net-zero by 2050. All pathways are also consistent with a rapid phase out of Russian gas imports in line with EU targets. Descriptions of each pathway are in Table 1.
Takeaways for Policymakers
1.0. Europe can achieve net-zero emissions by 2050 with a variety of potential energy mixes. Feasibility of each net-zero pathway depends on different economic, political, social, and technical risks.
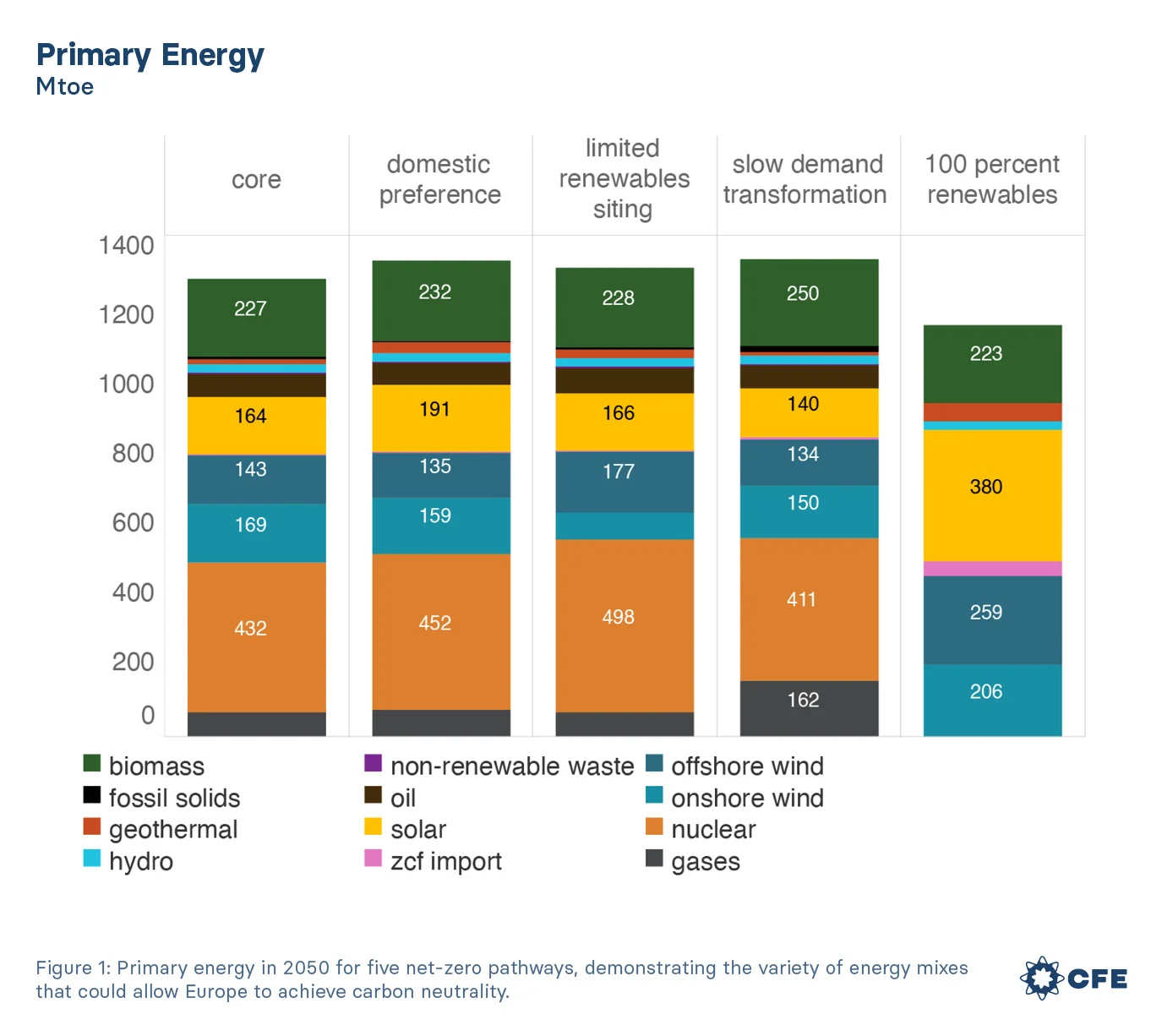
1.2. When nuclear power is allowed in the energy system (every pathway besides 100% Renewables), it becomes one of the largest sources of primary energy. When nuclear is not allowed due to countrylevel policy decisions, Europe would need almost a doubling of energy from wind and solar compared to other pathways.
1.3. Delays in electrification (Slow Demand Transformation) and a renewables-only strategy (100% Renewables) would both necessitate the import of zero-carbon fuels from outside of Europe. If not explicitly excluded, fossil fuels will remain a small part of a net-zero mix, mainly providing occasional power grid reliability and fueling some hard to decarbonise parts of the economy.
1.4. Shifting energy production to prefer domestic sources (Domestic Preference) does not significantly change the overall composition of energy supply compared to the Core pathway, but it does necessitate the deployment of lower-quality renewable resources that are accessible without additional transmission lines or pipelines to deliver hydrogen.
2.0. Risk assessment is an important framework to use when evaluating long-term energy plans. Required costs as well as pace and scale of infrastructure transformation are two of the largest risks to the feasibility of net-zero pathways. For more on risks of each net-zero pathway, read here.
2.1. The costs of the transition (including energy supply costs as well as demand-side equipment costs) borne by consumers differs by scenario. Using the Core pathway as a reference, we can see that slowing the pace of demand transformation, limiting the opportunities for siting renewables, limiting interregional delivery infrastructure like transmission lines and pipelines, and necessitating 100% renewables all increase the costs of achieving net-zero (Figure 2). A 100% renewables strategy is the most expensive scenario we evaluated, costing over €80 billion a year more by 2050 compared to the Core pathway.
2.2. Europe will need significant investments in clean energy technologies to reach carbon neutrality. Figure 3 shows the cumulative investments in different clean energy technologies for each pathway over the next three decades. While this is not a comprehensive list of investments needed to facilitate the net-zero transition, it does demonstrate where large investments should be targeted for transforming the power sector.
2.3. The pace of infrastructure deployment in each scenario can potentially be a limiting factor, with siting and permitting difficulties already inhibiting the development of key resources. Figure 4 shows the build rate of key electric sector technologies. Historically, the EU has built 23 gigawatts (GW) of wind and solar a year on average from 2011-2020. In our net-zero pathways, the EU will need to build on average between 27-79 GWs of solar annually, 6-25 GWs of onshore wind annually, and 11-22 GWs of offshore wind annually. Pathways that allow for a greater diversity in clean energy technologies have lower annual build rates.
3.0. The scale of electricity generation required to decarbonise Europe is enormous. Our model suggests that the amount of electricity generation will more than triple over the next 30 years as electrification of transport, heat, and hydrogen drives new demands for clean electricity.
3.1. The growth in electricity demand (Figure 5) places a large emphasis on the resource constraints Europe may ultimately face in terms of resource deployment. Gaining social acceptance for the scale and type of resource deployment necessary will ultimately be critical to achieving any of these decarbonisation pathways. The 100% Renewables pathway requires a quadrupling, rather than a tripling, of electricity generation to satisfy additional hydrogen production. Electrification will increase electricity load, even in the face of efficiency gains, though the scale of the system suggests that even more aggressive efficiency should be pursued than we model here.
3.2. As electricity becomes central to a larger part of the energy system, there will ultimately be a large emphasis on the reliability of the electricity system. Our modelling is able to maintain reliability of supply, even with an increasing share of overall energy coming from renewables, through the use of: battery storage; dispatchable thermal capacity such as advanced nuclear and thermal power plants that can run on low-carbon fuels to produce energy during periods of sustained low renewable generation; increased transmission interconnections to improve load and generation diversity; flexible end-use loads for thermal end-uses and electric vehicles; and opportunistic flexible loads like hydrogen electrolysis and hybrid boiler systems. Figure 6 shows each pathway’s mix of variable and firm, dispatchable resources for electric capacity to ensure reliability.
4.0. Decarbonisation changes the map of energy in Europe as the nature of energy resource endowments change. Whereas prior energy advantages included access to oil, natural gas, and coal resources, now resource advantages include: access to high quality renewable resources, biomass availability, geologic advantages for CO2 sequestration and hydrogen storage, and an acceptance of nuclear technology.
4.1. Current zero-carbon energy supply is dominated by countries with existing nuclear and hydro facilities with some additional contribution by countries with aggressive renewable deployment policies like Germany. In the future, countries will take advantage of their unique resource endowments to make contributions towards decarbonisation. Countries in Southern Europe deploy the vast majority of solar capacity; countries on the North Sea and Baltic Sea install most of the offshore wind; countries in northern and eastern Europe use their biomass resources to make advanced biofuels; and more countries leverage nuclear technology to produce energy in the absence of high-quality renewables (Figure 7).
4.2. Changing energy flows necessitate a buildout of delivery infrastructure including a large expansion of the existing transmission network as well as the buildout of a large backbone network of hydrogen pipelines (Figure 8).
5.0. Electrification of end-uses supported by the rapid and sustained deployment of renewables is responsible for a large fraction of the emissions reductions through 2030 and plays an important role to 2050. However, achieving net-zero emissions will require the deployment of other technologies.
5.1. Nuclear technology plays a complementary role to large-scale renewable deployment in the long-term. This includes maintaining existing nuclear facilities, building new nuclear facilities with current generation technology in the near-term, and deploying advanced nuclear technologies in the long-term. In addition to being a principal source of electricity generation in countries with more limited renewable resources, nuclear technology can lower the strain on renewable deployment necessary to support hydrogen production (by improving the efficiency of electrolysis (SOEC)), provide low-cost heat for direct air capture processes, and operate flexibly with the integration of thermal-energy storage to provide additional reliability services to the grid (Figure 9). It is also of increased importance to scenarios where there is difficulty siting renewables. There are additional potential applications of nuclear not yet explored in this analysis, including providing heat for industry, especially higher-temperature industrial processes for which there is not a viable non-fossil alternative outside of nuclear.
5.2. Carbon capture is also a required technology in all scenarios, though we do not see its deployment in the power sector. Instead, capture is utilised in biofuels production and industry (cement) with some scenarios also seeing a deployment of direct air capture facilities to offset residual emissions (Figure 10).
5.3. Captured carbon is either used to produce synthetic fuels (e-fuels) or stored underground to offset a small amount of continued fossil use (Figure 10). The balance of these approaches depends on additional policy constraints on fossil energy (i.e. 100% renewables), fossil fuel prices, the cost of hydrogen (as a feedstock for e-fuels), and the cost of geologic storage.
Technical Appendix
Click here to view the technical results, inputs, and assumptions of our modelling analysis.